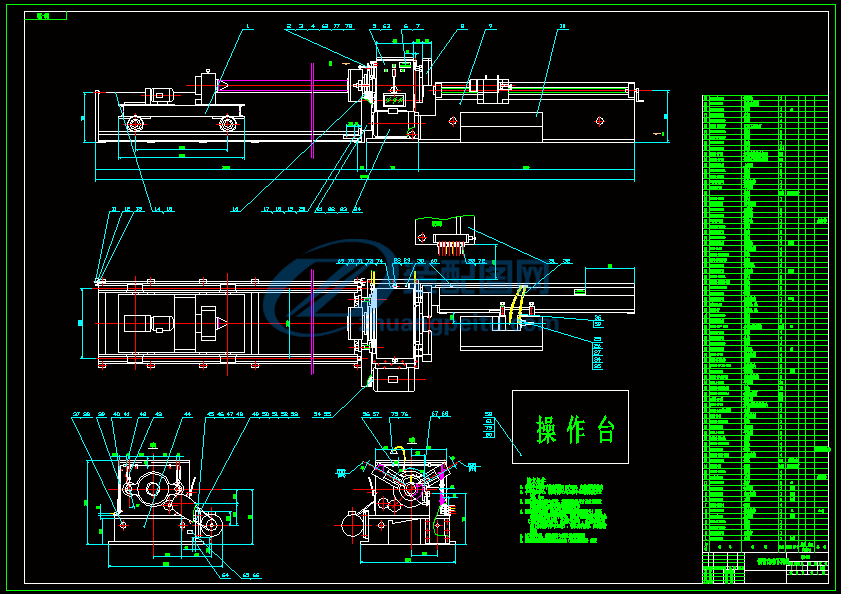
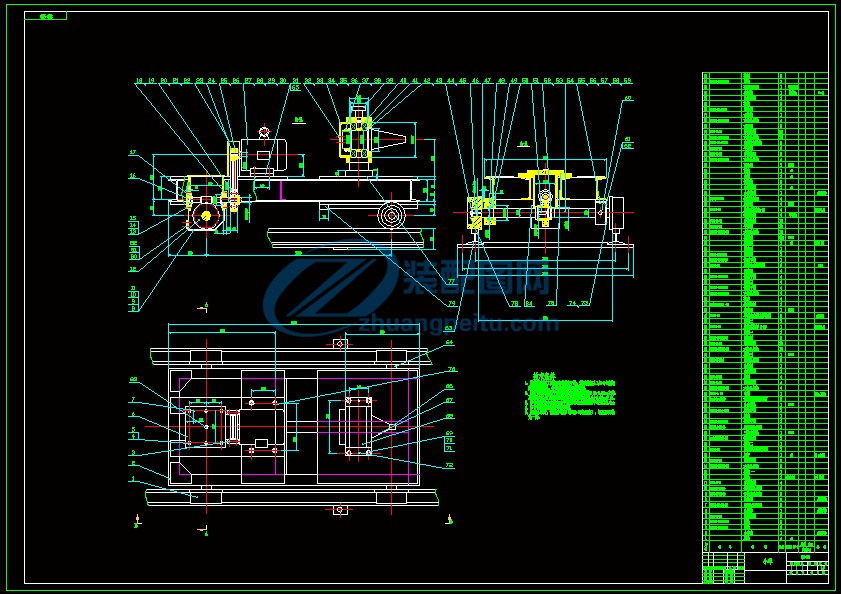
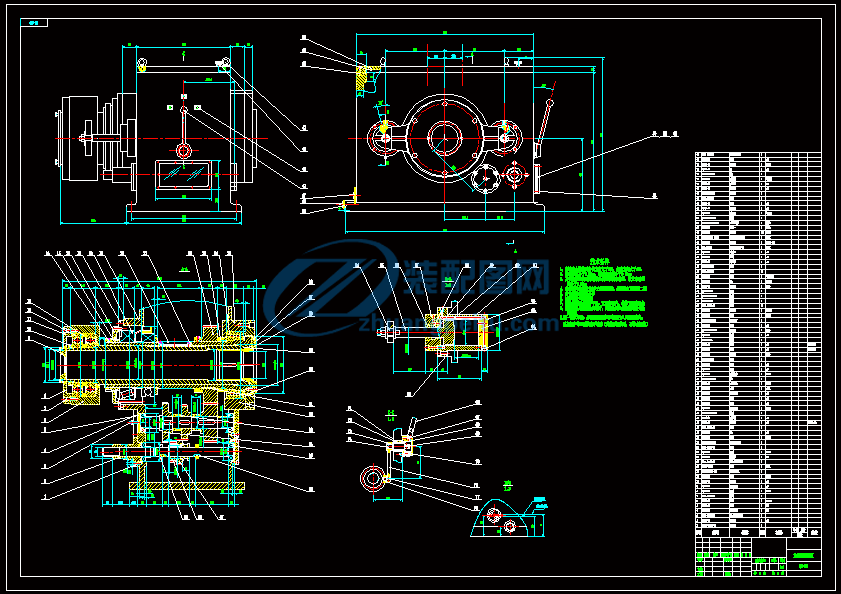
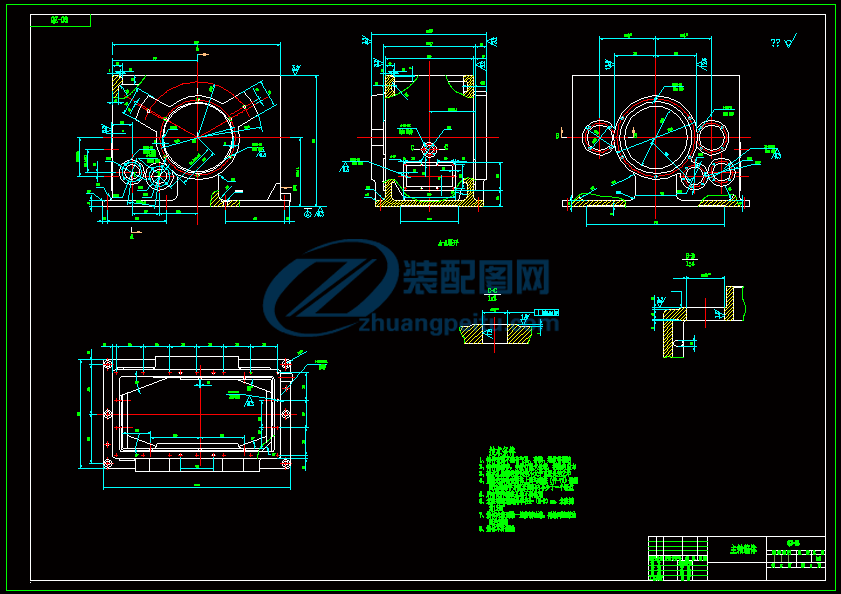
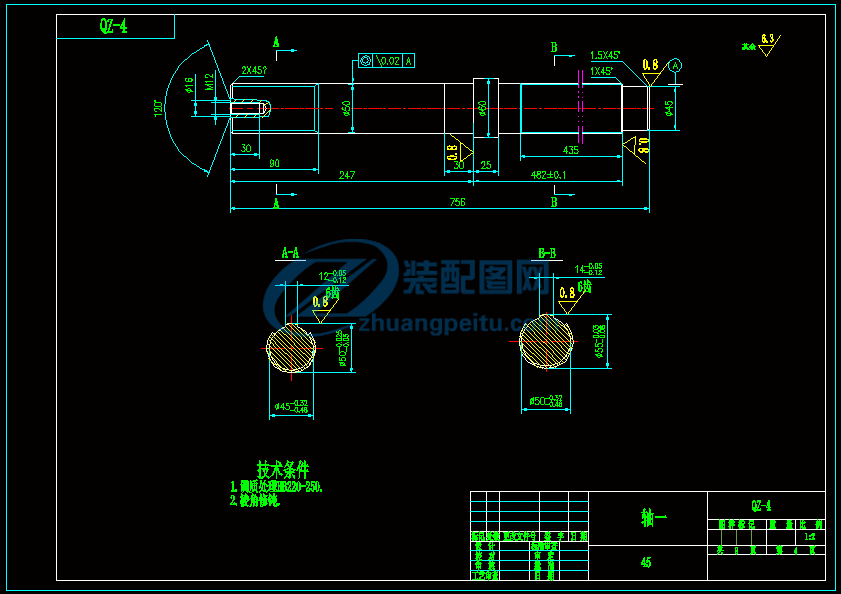
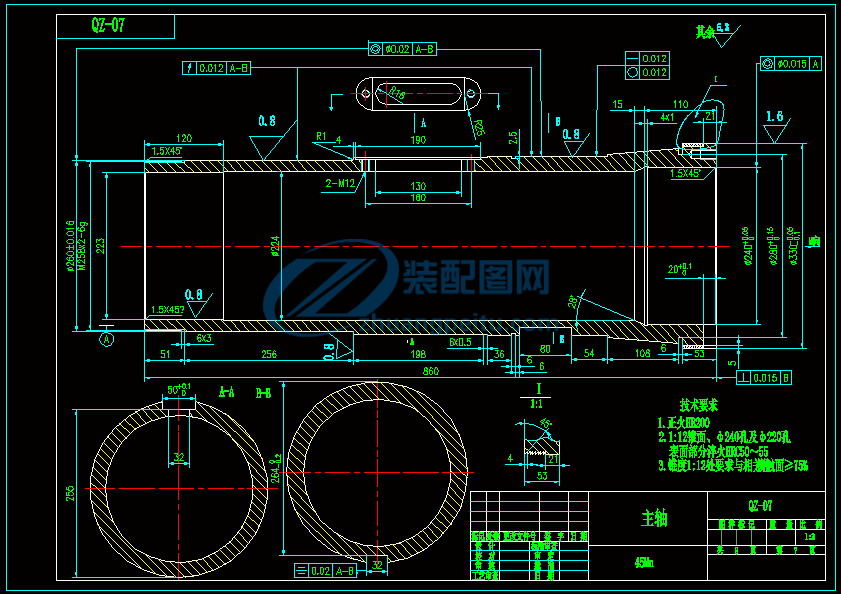
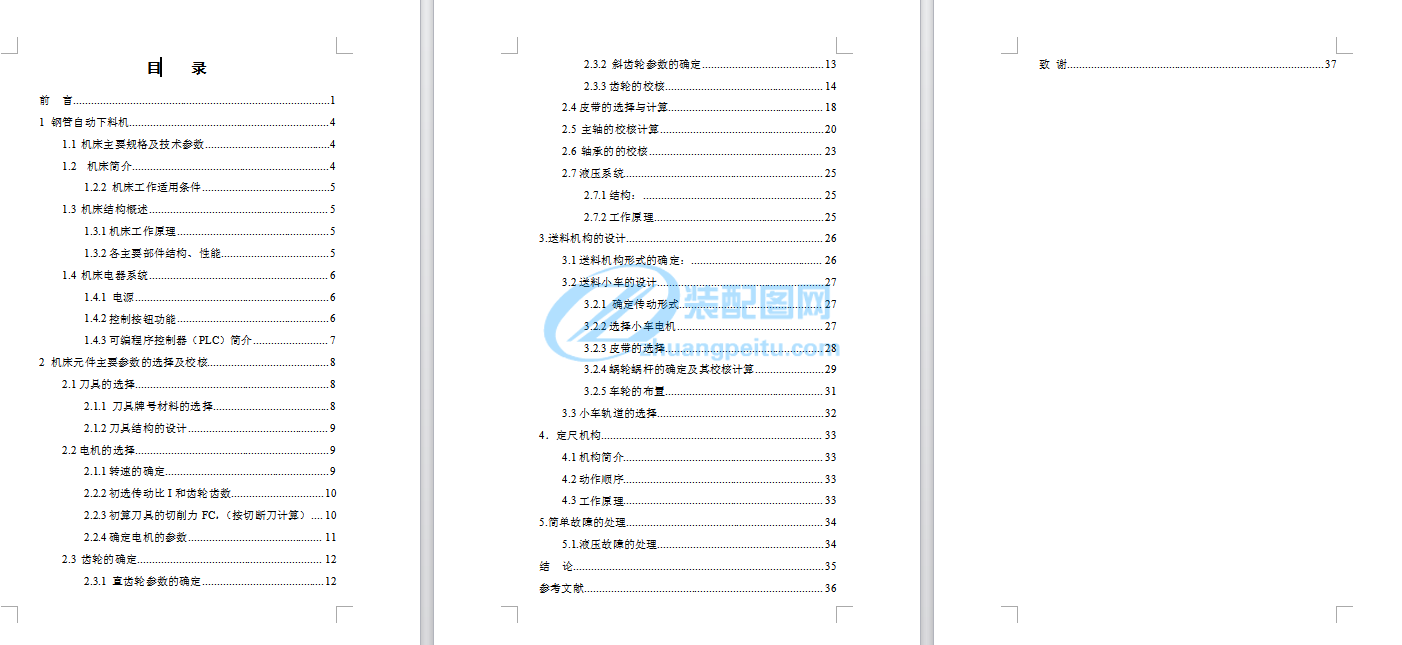
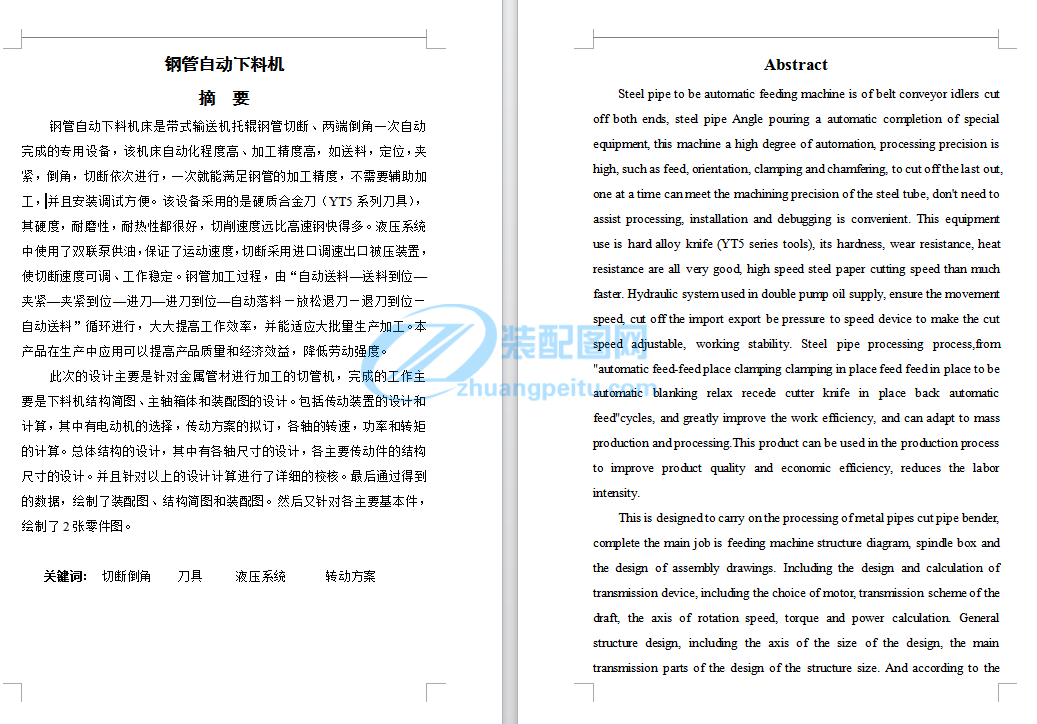
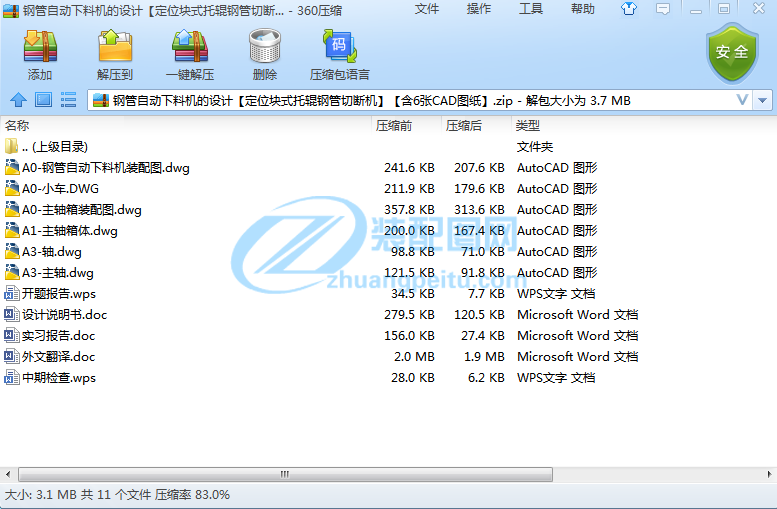
0英文原文Latest Developments in Belt Conveyor TechnologyM. A. AlspaughOverland Conveyor Co., Inc.Presented at MINExpo 2004Las Vegas, NV, USASeptember 27, 2004AbstractBulk material transportation requirements have continued to press the belt conveyor industry to carry higher tonnages over longer distances and more diverse routes. In order keep up, significant technology advances have been required in the field of system design, analysis and numerical simulation. Examples of complex conveying applications along with the numerical tools required to insure reliability and availability will be reviewed.IntroductionAlthough the title of this presentation indicates “new” developments in belt conveyor technology will be presented, most of the ideas and methods offered here have been around for some time. We doubt any single piece of equipment or idea presented will be “new” to many of you. What is “new” are the significant and complex systems being built with mostly mature components ,what is also “new” is the increasing ability to produce accurate computer simulations of system performance prior to the first system test (commissioning).As such, the main focus of this presentation will be the latest developments in complex system design essential to properly engineer and optimize today’s long distance conveyance requirements. The four specific topics covered will be:? Energy Efficiency? Route Optimization? Distributed Power? Analysis and Simulation1Energy EfficiencyMinimizing overall power consumption is a critical aspect of any project and belt conveyors are no different. Although belt conveyors have always been an efficient means of transporting large tonnages as compared to other transport methods, there are still various methods to reduce power requirements on overland conveyors. The main resistances of a belt conveyor are made up of:? Idler Resistance? Rubber indentation due to idler support? Material/Belt flexure due to sag being idlers? AlignmentThese resistances plus miscellaneous secondary resistances and forces to over come gravity (lift) make up the required power to move the material.In a typical in-plant conveyor of 400m length, power might be broken into its components as per Figure 1 with lift making up the largest single component but all friction forces making up the majority.In a high incline conveyor such as an underground mine slope belt, power might be broken down as per Figure 2, with lift contributing a huge majority. Since there is no way to reduce gravity forces, there are no means to significantly 2reduce power on high incline belts.But in a long overland conveyor, power components will look much more like Figure 3, with frictional components making up almost all the power. In this case, attention to the main resistances is essential.The specifics of power calculation is beyond the scope of this paper but it is 3important to note that significant research has been done on all four areas of idlers, rubber indentation, alignment and material/belt flexure over the last few years. And although not everyone is in agreement as to how to handle each specific area, it is generally well accepted that attention to these main resistances is necessary and important to overall project economics.At the 2004 SME annual meeting, Walter Kung of MAN Takraf presented a paper titled “The Henderson Coarse Ore Conveying System- A Review of Commissioning, Start-up and Operation”2. This project was commissioned in December 1999 and consisted of a 24 km (3 flight) overland conveying system to replace the underground mine to mill rail haulage system.The longest conveyor in this system (PC2) was 16.28 km in length with 475m of lift. The most important system fact was that 50% of the operating power (~4000 kW at 1783 mt/h and 4.6 m/s) was required to turn an empty belt therefore power efficiency was critical. Very close attention was focused on the idlers, belt cover rubber and alignment. One way to document relative differences 4in efficiency is to use the DIN 22101 standard definition of “equivalent friction factor- f” as a way to compare the total of the main resistances. In the past, a typical DIN fused for design of a conveyor like this might be around 0.016. MAN Takraf was estimating their attention to power would allow them to realize an f of 0.011, a reduction of over 30%. This reduction contributed a significant saving in capital cost of the equipment. The actual measured results over 6 operating shifts after commissioning showed the value to be 0.0075, or even 30% lower than expected. Mr. Kung stated this reduction from expected to result in an additional US$100, 000 savings per year in electricity costs alone.Route OptimizationHorizontal AdaptabilityOf course the most efficient way to transport material from one point to the next is as directly as possible. But as we continue to transport longer distances by conveyor, the possibility of conveying in a straight line is less and less likely as many natural and man-made obstacles exist. The first horizontally curved conveyors were installed many years ago, but today it seems just about every overland conveyor being installed has at least one horizontal change in direction. And today’s technology allows designers to accommodate these curves relatively easily.Figures 5 and 6 shows an overland conveyor transporting coal from the 5stockpile to the ship loader at the Tianjin China Port Authority installed this year. Designed by E.J.O’Donovan however the system engineer can seldom test the finished system until it is completed on site. Therefore computational methods and tools are absolutely critical to simulate the interactions of various diverse disciplines and components. Dynamic Starting and StoppingWhen performing starting and stopping calculations per CEMA or DIN 22101 (static analysis), it is assumed all masses are accelerated at the same time and rate; in other words the belt is a rigid body (non-elastic). In reality, drive torque transmitted to the belt via the drive pulley creates a stress wave which starts the belt moving gradually as the wave propagates along the belt. Stress 15variations along the belt (and therefore elastic stretch of the belt) are caused by these longitudinal waves dampened by resistances to motion as described above.Many publications since 1959 have documented that neglecting belt elasticity in high capacity and/or long length conveyors during stopping and starting can lead to incorrect selection of the belting, drives, take-up, etc. Failure to include transient response to elasticity can result in inaccurate prediction of:? Maximum belt stresses? Maximum forces on pulleys? Minimum belt stresses and material spillage? Take-up force requirements? Take-up travel and speed requirements? Drive slip? Breakaway torque? Holdback torque? Load sharing between multiple drives? Material stability on an inclineIt is, therefore, important a mathematical model of the belt conveyor that takes belt elasticity into account during stopping and starting be considered in these critical, long applications. A model of the complete conveyor system can be achieved by dividing the conveyor into a series of finite elements. Each element has a mass and rheological spring as illustrated in Figure 26. Many methods of analyzing a belt’s physical behavior as a rheological spring have been studied and various techniques have been used. An appropriate model needs to address:1. Elastic modulus of the belt longitudinal tensile member2. Resistances to motion which are velocity dependent (i.e. idlers)3. Viscoelastic losses due to rubber-idler indentation4. Apparent belt modulus changes due to belt sag between idlers 16unloading. The transfer chute is often sited as the highest maintenance area of the conveyor and many significant production risks are centered here.Since the mathematics necessary to solve these dynamic problems are very complex, it is not the goal of this presentation to detail the theoretical basis of dynamic analysis. Rather, the purpose is to stress that as belt lengths increase and as horizontal curves and distributed power becomes more common, the importance of dynamic analysis taking belt elasticity into account is vital to properly develop control algorithms during both stopping and starting.Using the 8.5 km conveyor in Figure 23 as an example, two simulations of starting were performed to compare control algorithms. With a 2x1000 kW drive installed at the head end, a 2x1000 kW drive at a midpoint carry side location and a 1x1000kW drive at the tail, extreme care must be taken to insure proper coordination of all drives is maintained.Figure 27 illustrates a 90 second start with very poor coordination and severe oscillations in torque with corresponding oscillations in velocity and belt tensions. The T1/T2 slip ratio indicates drive slip could occur. Figure 28 shows the corresponding charts from a relatively good 180 second start coordinated to safely and smoothly accelerate the conveyor.Mass Flow at Transfer PointsOne of the reasons for using intermediate drives and running single flight conveyors longer and longer is to eliminate transfer points. Many of the most difficult problems associated with belt conveyors center around loading and? Plugging? Belt and Chute Damage and Abrasion? Material Degradation? Dust? Off Center Loading/SpillageIn the past, no analytical tools have been available to the design engineer so trial-and-error and experience were the only design methods available. Today, numerical simulation methods exist which allow designers to “test” their design prior to fabrication. 1819Numerical simulation is the discipline of designing a model of an actual physical system, executing the model on a computer, and analyzing the results.Simulation embodies the principle of “l(fā)earning by doing''. To understand reality and all of its complexity, we build artificial objects in the computer and dynamically watch the interactions.The Discrete Element Method (DEM) is a family of numerical modeling techniques and equations specifically designed to solve problems in engineering and applied science that exhibit gross discontinuous mechanical behavior such as bulk material flow. It should be noted that problems dominated by discontinuum behavior cannot be simulated with conventional continuum based computer modeling methods such as finite element analysis, finite difference procedures and/or even computational fluid dynamics (CFD).The DEM explicitly models the dynamic motion and mechanical interactions of each body or particle in the physical problem throughout a simulation and provides a detailed description of the positions, velocities, and forces acting on each body and/or particle at discrete points in time during the analysis. In the analysis, particles are modeled as shaped bodies. The bodies can interact with each other, with transfer boundary surfaces and with moving rubber conveyor belt surfaces. The contact/impact phenomena between the interacting bodies are modeled with a contact force law which has components defined in the normal and shear directions as well as rotation. The normal contact force component is generated with a linear elastic restoring component and a viscous damping term to simulate the energy loss in a normal collision. The linear elasticcomponent is modeled with a spring whose coefficient is based upon the normal 20stiffness of the contact bodies and the normal viscous damper coefficient is defined in terms of an equivalent coefficient of restitution (Figure 29).Figure 30 shows particles falling through a transfer chute. The colors of the particles in the visualization represent their velocity. The RED color is zero velocity while BLUE is the highest velocity. Perhaps the greatest benefit that can be derived form the use of these tools is the feeling an experienced engineer can develop by visualizing performance prior to building. From this feel, the designer can arrange the components in order to eliminate unwanted behavior. Other quantitative data can also be captured including impact and shear forces (wear) on the belt or chute walls.FutureBigger Belt ConveyorsThis paper referenced Henderson PC2 which is one of the longest single flight conventional conveyors in the world at 16.26 km. But a 19.1 km conveyor is under construction in the USA now, and a 23.5 km flight is being designed in Australia. Other conveyors 30-40 km long are being discussed in other parts of 21the world. Belt manufacturers have developed low rolling resistance rubber with claims of 10-15% power savings as methods to quantify indentation have become known. Together with improved installation methods and alignment, significant power efficiencies are possible.Underground coal mines and tunneling contractors will continue to use the proven concept of distributed power to their best advantage, but now at least two of the longer surface conveyors in development will be installing intermediate drives in 2005.In Germany, RWE Rheinbraun operates coal conveyors with 30,000 tph capacities and other surface coal mines have plans to soon be approaching these loads. With capacity increases, comes increases in belt speed; again demanding better installation, manufacturing tolerances and understanding of resistances and power. Each time we go longer, higher, wider or faster, we stretch the limits of our analytical tools to predict system performance. And because each conveyor is unique, the only way we have to predict performance is our numerical analysis and simulation tools. Therefore it is imperative we continue to improve our design tools as our goals get bigger. Belt Conveyors for Bulk Materials, 6th Ed, CEMAThe Conveyor Equipment Manufacturers Association (CEMA), recognizing many of the trends discussed in this paper, is currently producing the 6th Edition of the worldwide reference manual “Belt Conveyors for Bulk Materials” with longer center conveyors in mind. This is the first major revision of this manual since the 1980’s and reflects the need to update design methods for today’s demanding applications.22中文譯文輸送帶技術(shù)的最新發(fā)展M. A. AlspaughOverland Conveyor Co., Inc.Presented at MINExpo 2004Las Vegas, NV, USASeptember 27, 2004摘要大量的物質(zhì)運輸需求在促使帶式輸送機繼續(xù)朝大運量、長距離、多路徑發(fā)展。為滿足生產(chǎn)力的發(fā)展,在系統(tǒng)設(shè)計、分析和數(shù)值模擬等領(lǐng)域需要更多重要的技術(shù)革新。在這里我們將回顧一些復(fù)雜的、利用數(shù)學工具來保證其可靠性和適用性的實例。緒論盡管這篇文章的標題指出將會展現(xiàn)給大家?guī)捷斔蜋C“新的”發(fā)展技術(shù),但當中的大多數(shù)的思想和方法早已經(jīng)四處流傳了。恐怕其中任何一個的簡單設(shè)備或想法對大多數(shù)人都不是“新的”。 所謂“新的”東西就是一個在成熟的條件下建立的復(fù)雜、重要的系統(tǒng),是在一個系統(tǒng)檢測(試運轉(zhuǎn))之前對系統(tǒng)性能的精確計算機模擬能力的提高。因而,我們討論的主要焦點是,復(fù)雜帶式輸送機系統(tǒng)設(shè)計的最新發(fā)展,以及針對長距離運輸?shù)脑O(shè)計和優(yōu)化。四個被涵蓋的主題是:? 能量利用效率? 線路優(yōu)化? 動力分配? 分析和仿真能量利用率將總耗電量降到最小不論對于帶式輸送機還是其它項目都是問題的關(guān)鍵。盡管地面大運量運輸時利用帶式輸送機總是效率很高的手段,還是有23很多方法來降低其能量需求。帶式輸送機的運行阻力由以下幾部分組成:? 托輥阻力? 膠帶與托輥架擠壓? 膠帶垂度的影響? 調(diào)心托輥的影響這些阻力加上其它各種次要的阻力以及用來克服重力的升力,就形成所需總共的動力。在一條 400 m 長度的典型廠內(nèi)輸送機中,動力可能依照圖 1 被分成幾部分,其中運輸提升所需的動力是其中最大的一塊,而所有摩擦阻力又占它的了多半數(shù)。對于大傾角輸送機, 例如煤礦井下帶式輸送機,動力可能依照圖 2 所示分配,其中用于提升的動力占了極大的一部分。 因為沒有方法減少重力的影響,所以就沒有方法能顯著地減少在大傾角帶式輸送機上的動力消耗。但是在長距離地面輸送機中,動力組成的幾個部分就與圖3所示較為相似,動力幾乎全都消耗在摩擦阻力上。所以在這種情況下應(yīng)特別關(guān)注帶式輸送機的主要阻力。有關(guān)詳細的動力計算在這里不做贅述,但值得關(guān)注的是,對于托輥、膠帶、調(diào)心、物料/膠帶彎曲這四方面近年來已完成許多有意義的研究工作。對于各個具體方面的問題如何處理,雖然仁者見仁、智者見智,但通常大家都認為,將著眼點放在主要阻力上對整個工程的經(jīng)濟性是重要且必需的。在 2004 SME 年會上,MAN Takraf 的Walter·Kung 介紹了一篇題為“亨德森粗礦石運輸系統(tǒng)——運行、啟動、操作評估”的論文。 這個工程于 1999 年 12月啟動,是由一條 24公里(3段)的地面輸送系統(tǒng)來代替地下挖掘碾磨軌道拖運系統(tǒng)。這個系統(tǒng) (PC2) 中的最長運送機長16.28 公里,提升高度475 m 。系統(tǒng)最重要的工況是使帶空載運轉(zhuǎn)時需要50% 的額定功率(約4000 千瓦,在1783 mtph 和 4.6 m/s時 ),這時功率效率達到臨界值。要把注意力集中在托輥, 覆蓋膠和調(diào)心上。 一種判斷效率上的相對差異的方法是使用 DIN(德國工業(yè)標準) 22101 標準定義中的“ 等效磨擦因數(shù)—f“ ,就如比較各個主要阻力的相對差異的方法一樣。過去,用于設(shè)計輸送機的典型f值DIN規(guī)定為0.016左右,但 MAN Takraf 估計以他們對動力情況的了解,允24許他們將f值降低30%,達到0.011。該值的減少會給設(shè)備的基本投資節(jié)省一筆不小的開支。試運行后,6次換檔的實測結(jié)果顯示,f值為0.0075,或是更小,比期望值低30%左右。 Kung說,只將f從期望值減少每年就會節(jié)省電費$100,000。路徑最優(yōu)化水平運輸?shù)倪m用性當然,從一處到另一處運送物料最有效的方法是盡可能直接地運輸。但是當我們用輸送機長距離連續(xù)輸送時,由于人為或自然的原因,能在直線運輸?shù)目赡苄陨僦稚佟5谝慌_水平曲線式輸送機的安裝在數(shù)年以前,但是今天幾乎每一臺安裝的地面輸送機都至少有一個水平方向的變化。而且今天的技術(shù)允許設(shè)計者相對容易地調(diào)整這些曲線。 圖 5 和 6 顯示在中國天津港務(wù)局,一條今年安裝的地面帶式輸送機正將煤從倉庫運送到裝船機。 這條9 公里的帶式輸送機由 E.J.O'Donovan與 Associates 設(shè)計、澳大利亞的Continental Conveyor 公司制造,運量為6000 mtph ,驅(qū)動功率為4 x1500 kW。位于美國懷俄明州Powder River 盆地的Wyodak礦井,是自1923年美國有年產(chǎn)量記錄以來最老的煤礦,而且如今還在不斷地產(chǎn)煤。它現(xiàn)在利用一臺水平半徑為700 m(2,300 ft) 地面帶式輸送機(圖 7) ,新煤坑離廠 756 m (2,482 英尺)。這證明受益于水平轉(zhuǎn)向,帶式輸送機不需要非常的長。隧道如果沒有水平曲線,建造隧道就不能利用帶式輸送機了。全世界都在挖掘隧道,以作為下水道或是運輸?shù)然A(chǔ)設(shè)施。將挖掘隧道時產(chǎn)生的棄渣運出,最有效的方法是在隧道鉆機的尾部連接一個推進式帶式輸送機。但很少有隧道是筆直的。例如,西班牙巴塞羅那的地下有一條直徑為 10.9 m 隧道,它是倫敦地鐵系統(tǒng)的延伸。Continental Conveyor 公司安裝了如圖 8 、9所示的第一個4.7 公里輸送機,而且最近已經(jīng)接受負責安裝第二個 8.39 公里的輸送機。Frontier Kemper Construction 正開始為Metropolitan St. Louis (位于密蘇里州)的排水區(qū)挖掘一條6.18 公里(20,275 英尺) 長、3.6 m(12英尺)寬的隧道。 這個名為Baumgartner 的隧道(圖 10) 將會裝備一個長6.1公里、帶寬600 毫米、具有4個中間傳動裝置的帶式輸送機。管狀輸送機25如果傳統(tǒng)的輸送機不能夠滿足半徑的要求, 就可用其他帶式運送機的變體例如管狀帶式輸送機。簡單的描述一下,一個管狀輸送機由一個卷成桶狀的傳送帶與輥子組成。這種很基本的設(shè)計方法可以使物料被輸送帶完全包起來,直接產(chǎn)生較高的效益。托輥在各方向約束著膠帶,就允許膠帶朝各個方向較大地彎曲。 曲線可能是水平的,垂直的或二者的結(jié)合。而傳統(tǒng)的輸送機只能靠膠帶和托輥的重力和摩擦力保證運輸路徑。管狀輸送機的另一個好處是能減少粉塵及灑漏,因為物料是完全被封閉的。這有一個經(jīng)典的例子,在美國猶他州的Skyline 礦中(圖 12),路徑與周圍環(huán)境很好的適應(yīng). 這條3.38 公里(11,088 英尺)長的管狀輸送機是由ThyssenKrupp Robins 安裝,穿過一個國家森林,而且中間水平換向22次、豎直換向45次。Metso鋼繩輸送機Metso鋼繩輸送機(MRC)是另一種從傳統(tǒng)型變化而來輸送機,人們一般稱它為鋼繩牽引輸送機。這一產(chǎn)品以長距離輸送聞名,世界最長的刮板式輸送機在澳大利亞的 Worsley Alumina ,長度為 30.4千米。它的牽引體與承載體是分開的(圖 13)。垂直的適用性有時物質(zhì)的需要被升起或降落,而常規(guī)的輸送機的傾角被限制在16-18 度。 但是帶式輸送機的再一次突破常規(guī)的變化使帶式輸送機可以成功地增大傾角,甚至可以垂直輸送。大傾角輸送機 (HAC)大傾角輸送機的第一臺樣機由Continental Conveyor 換句話說,帶一個剛體(如泡泡)。事實上,傳送到皮帶驅(qū)動力矩通過驅(qū)動滑輪創(chuàng)造了一個應(yīng)力波帶逐漸開始移動的雷達波沿腰帶。應(yīng)力沿著帶變化(因此彈性伸展帶)是由于這些縱向波運動絲毫抗逆性如上所述。自 1959 年以來有許多出版物上記載,當時忽略帶彈性高容量的輸送和/28或長在停止和啟動會導(dǎo)致不正確的選擇、驅(qū)動器、卷取機膠帶等。失敗,包括彈性瞬態(tài)響應(yīng)的預(yù)測會導(dǎo)致不準確。最大混濁帶應(yīng)力最大受力滑輪最低帶應(yīng)力和材料泄漏或溢出收力要求收旅行和速度的要求驅(qū)動滑分離的扭矩扭矩蓄負載共享之間的多重驅(qū)動器材料穩(wěn)定性在一個斜坡因此,重要的數(shù)學模型,以帶彈性帶式輸送機在開始考慮停止,并被認為是在當前的危機,長期應(yīng)用。一個完整的系統(tǒng)模型能夠?qū)崿F(xiàn)輸送機輸送帶劃分成一系列有限元素。每個元素都有一個質(zhì)量和流變春天如圖 26 歲。許多措施來分析一個帶的物理行為進行了研究流變春天和各種不同的技術(shù)已被使用。一個合適的模式需要地址:1 彈性模量皮帶縱向拉伸成員2 運動的抗逆性(即托輥速度依賴)3 粘彈性損失縮進4 由于模量變化明顯帶帶凹陷托輥之間卸載。瀑布附近轉(zhuǎn)移通常是為最高維護地區(qū)的許多重大生產(chǎn)輸送機和風險都集中在這里。從數(shù)學必要從解決這些動態(tài)問題非常復(fù)雜,它不是這樣做的目的詳細報告的理論基礎(chǔ)進行動態(tài)分析。更確切地說,這樣做的目的是要強調(diào)的是,皮帶長度增加,如水平曲線和分布式電源變得越來越普遍的重29要性,以帶彈性動力學分析考慮適當發(fā)展是至關(guān)重要的控制算法在停止和啟動。使用 8.5 公里輸送機在圖 23 為例,進行了兩個模擬比較開始控制算法。以 2 千瓦 x1000 安裝驅(qū)動的在頭結(jié)束,一個 2 x1000 千瓦開車帶在身邊中點位置和一個 1 x1000kW 尾巴,極端的速度駕車必須注意確保適當?shù)膮f(xié)調(diào)所有驅(qū)動器被維持。圖 27 也說明了一個 90 年的二開始艱難困苦的協(xié)調(diào)和嚴重的振蕩扭矩與相應(yīng)的振蕩速度和帶間的緊張關(guān)系。T1 / T2 滑移率的顯示驅(qū)動滑移會出現(xiàn)。圖 28 顯示相應(yīng)的圖表從一個比較好的調(diào)整到 180 第二次首發(fā)安全、正常加速輸送機。在傳輸點質(zhì)量流量原因之一中間驅(qū)動和運行使用單航班輸送機加長,消除傳輸點。許多最困難的有關(guān)的問題,圍繞負荷和帶式輸送機堵帶、溜槽傷害和磨損材料劣化灰塵離中心加載/溢出在過去,沒有分析工具已經(jīng)在設(shè)計工程師所以試誤和經(jīng)驗是唯一可用的設(shè)計方法。今天,數(shù)值模擬方法存在使設(shè)計者最為人們熟知的“測試”之前,他們的設(shè)計制造。數(shù)值仿真設(shè)計學科的一個實際的物理系統(tǒng)模型,該模型在一臺計算機上執(zhí)行,分析測試結(jié)果。模擬的原則體現(xiàn)了“從做中學” 。理解現(xiàn)實和它所有的復(fù)雜性,我們制造人工物體在電腦和動態(tài)觀察之間的相互作用。離散單元法(DEM)是一個家庭的數(shù)值模擬技術(shù)和方程專門設(shè)計來解決問題,在工程與應(yīng)用科學學院展現(xiàn)總不連續(xù)等力學行為,松散材料的流動。應(yīng)該指出的是,問題 discontinuum 占主導(dǎo)地位的行為不能與傳統(tǒng)的基于計算機模擬連續(xù)建模方法如有限元分析、有限差分程序和/或甚至計算流體動力學(CFD)。30其離散元模型的動態(tài)運動和明確的每一個身體或機械相互作用粒子的整個仿真和實際問題提供了詳細的描述和位置,速度,力量作用于身體的每個和/或離散時間點的粒子在分析。在分析、粒子形狀建模為身體。尸體可以互相作用,表面采用傳輸和移動邊界橡膠輸送帶的表面。接觸/沖擊現(xiàn)象之間的相互作用進行建模與身體接觸力法,定義了在正常元件和剪切方向以及旋轉(zhuǎn)。正常的接觸力構(gòu)件產(chǎn)生線性彈性恢復(fù)和一種粘性的組件阻尼項來模擬一個正常的碰撞能量損失。線性彈性以彈簧部件的系數(shù)是基于正常接觸物體的剛度和正常的粘性阻尼系數(shù)的角度來定義的等效系數(shù)歸還(如圖 29)。圖 30 顯示顆粒沉降槽通過轉(zhuǎn)移。粒子的顏色的可視化表達自己的速度。紅顏色是零速而藍色的最高速度。或許最大的好處,可以來源于使用這些工具是一位有經(jīng)驗的工程師的感覺能開發(fā)前觀察表現(xiàn)建筑。從這感覺,設(shè)計師可以安排組件以消除不必要的行為。其他定量數(shù)據(jù)也可以包括影響捕獲和剪切力(磨損)在帶或瀑布墻。未來更大的帶式輸送機的本文引用,亨德森 PC2 最長的一個單航班常規(guī)式輸送機在世界全長 16.26 公里。但是 19.1 公里的輸送機正在建設(shè)中在美國現(xiàn)在,和 23.5 公里的航班是被設(shè)計成在澳大利亞。其他輸送帶,30 - 40 公里長正在討論之中在世界上的其它地方。皮帶生產(chǎn)商已經(jīng)開發(fā)出低滾動阻力橡膠請求 10 - 15%的節(jié)省電源的方法已經(jīng)知道了量化縮進。用改進后的安裝方法和一起對齊、強大的力量效率是可能的。地下煤礦掘進承包商將繼續(xù)使用分布式電源的概念的證明他們的優(yōu)勢,但是現(xiàn)在至少有兩個更長時間的表面式輸送機在發(fā)展中間驅(qū)動安裝在 2005年。在德國,Rheinbraun RWE 經(jīng)營煤 30000 tph 輸送能力和其他表面煤礦計