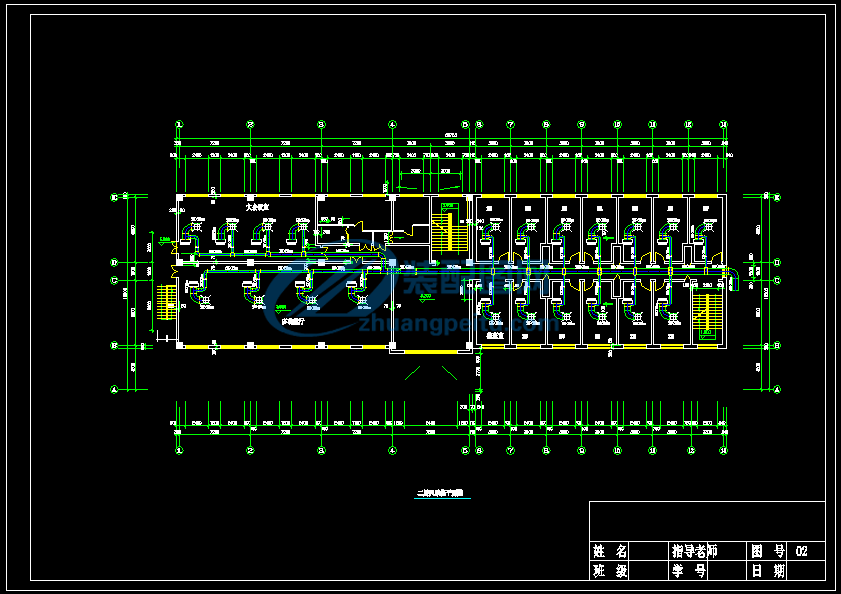
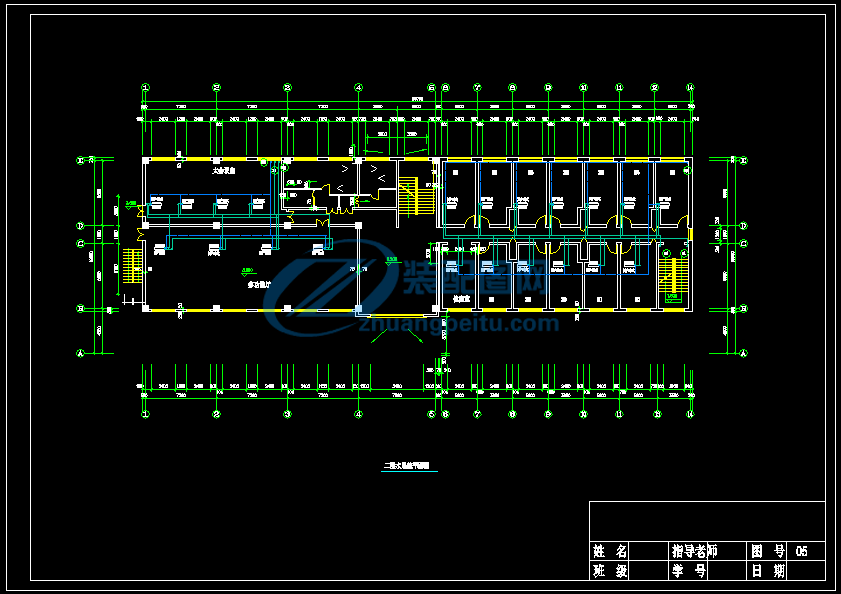

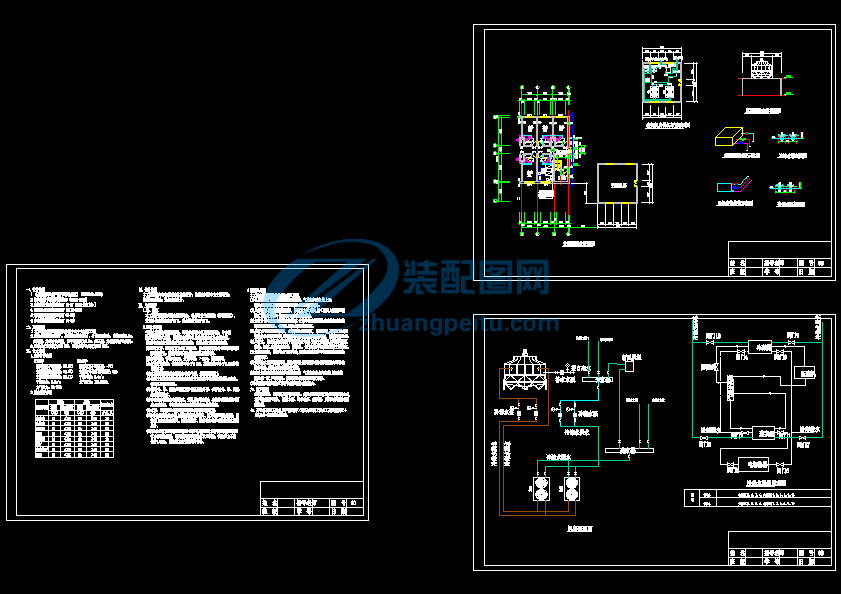


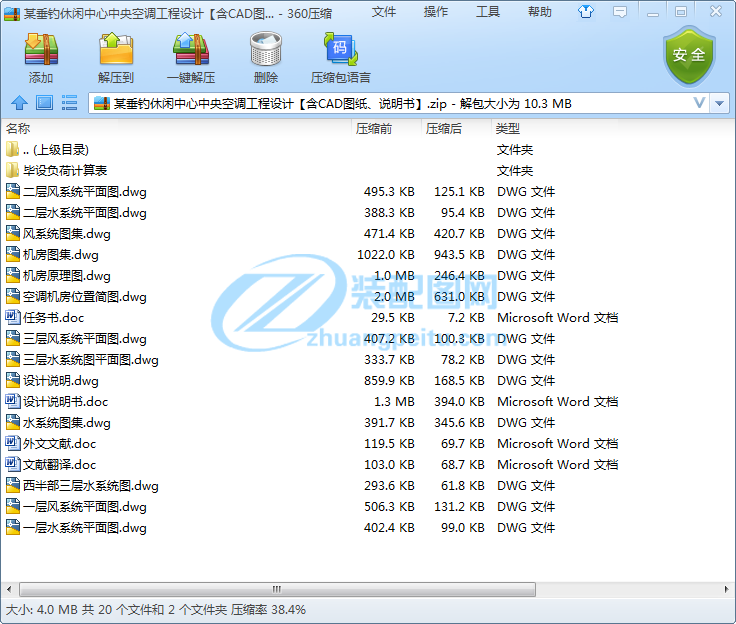
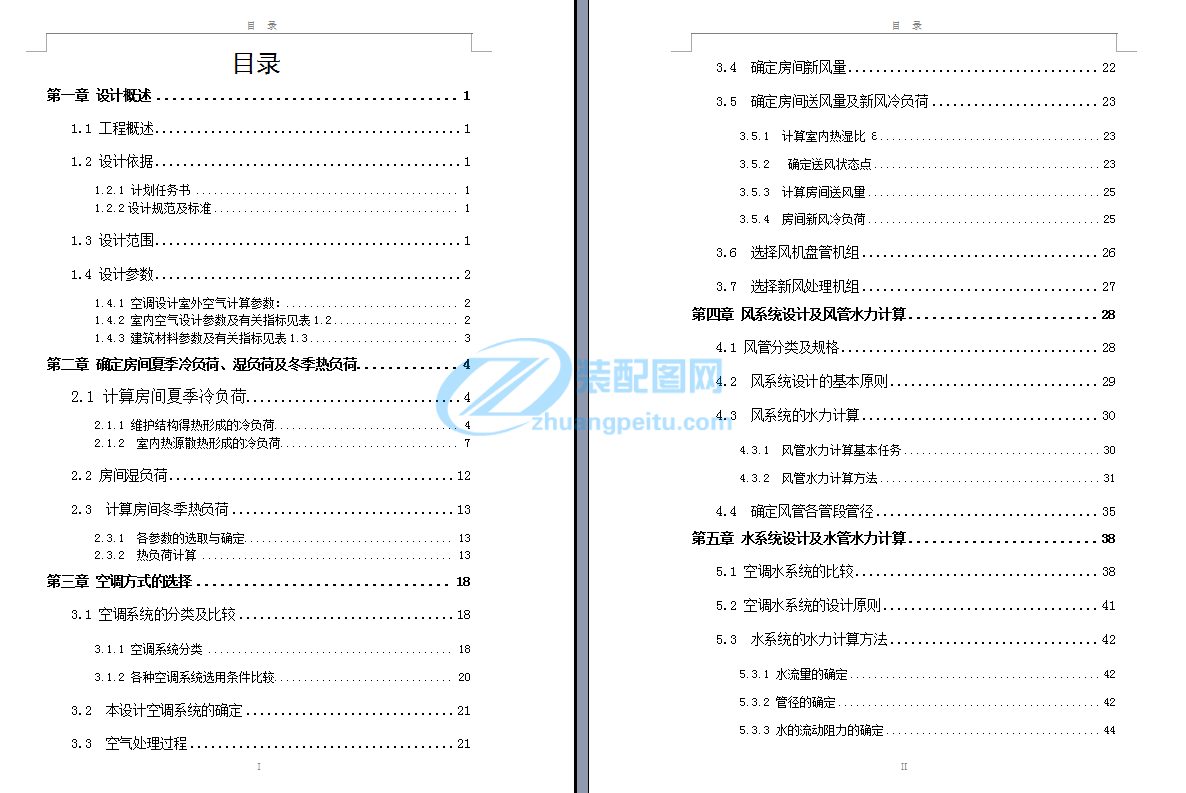

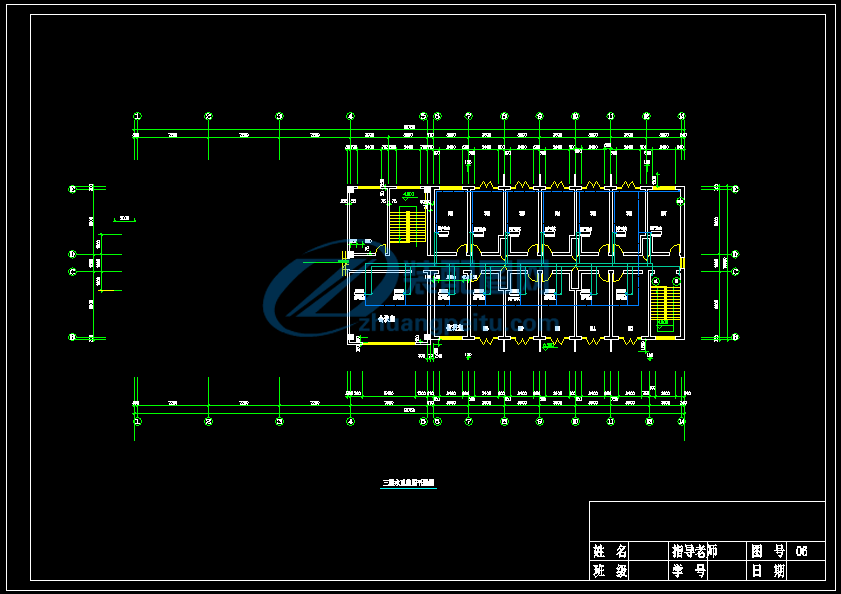
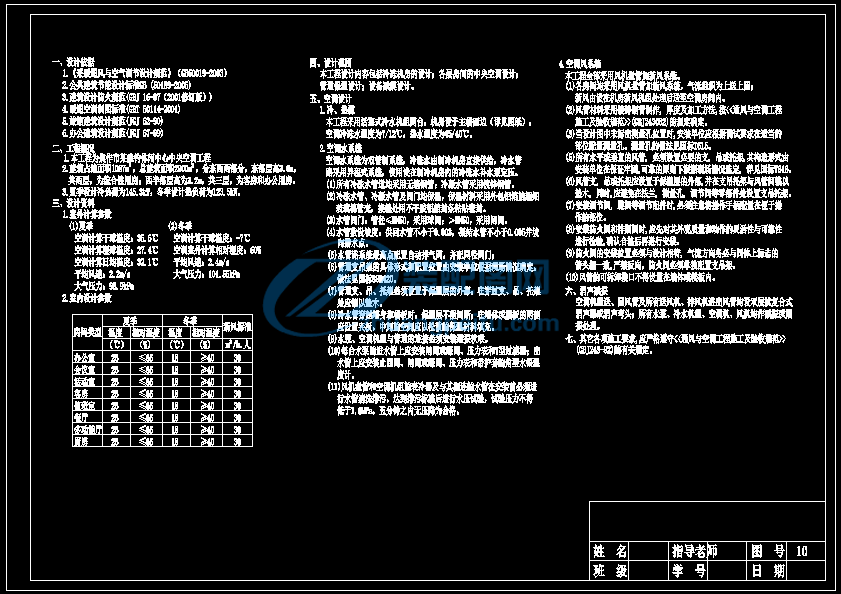
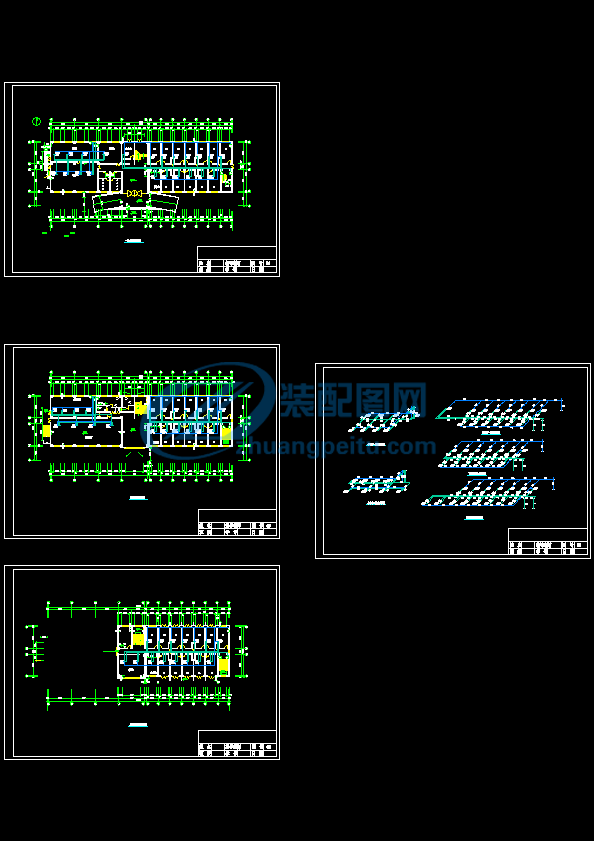
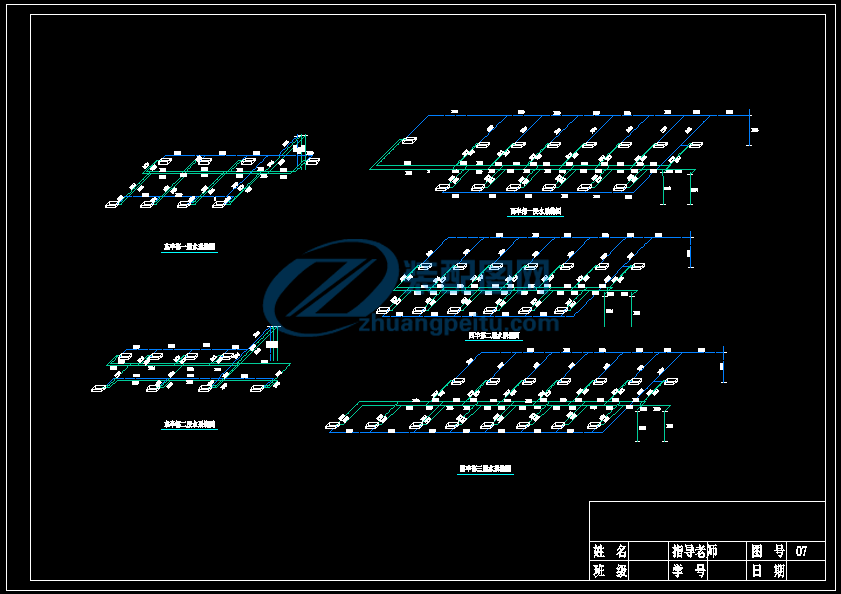
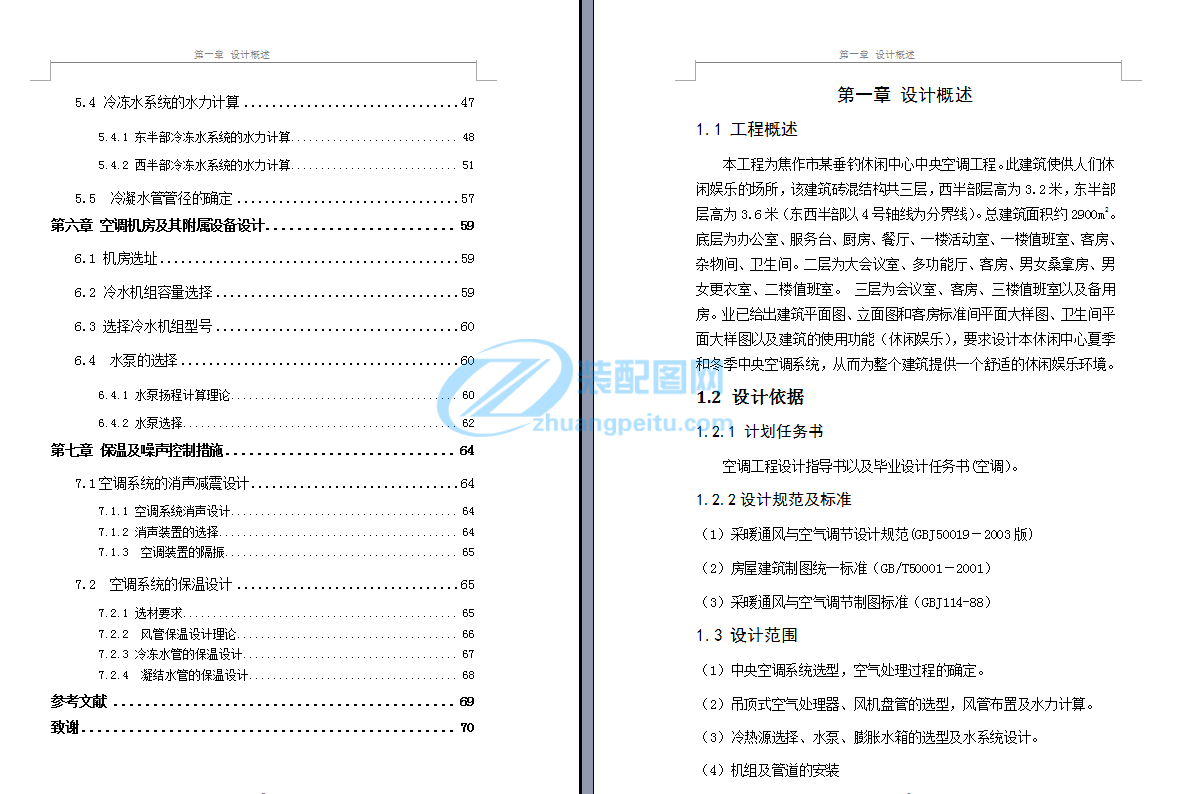
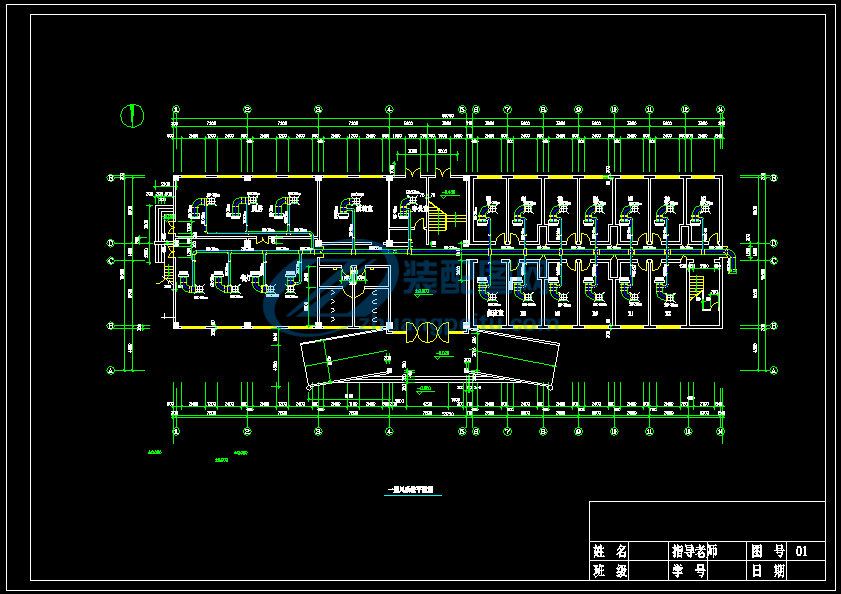
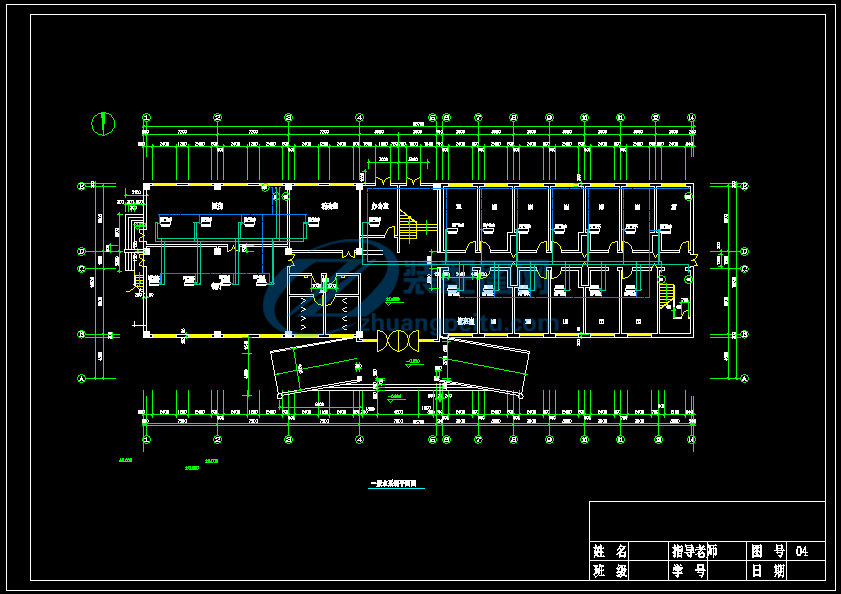
Development and Encouragement of renewable energy technologies1.1Project BackgroundSince its inception in the 1970s, the U.S. Department of Energy (DOE) has operated a substantial program in the development and encouragement of renewable energy technologies. As part of its ongoing effort to document the status and potential of these technologies, DOE, along with its national laboratories and support organizations, developed the first set of Renewable Energy Technology Characterizations (TCs) in 1989. The TCs were designed to respond to DOE’s need for a set of consistent cost and performance data to support the development of the biennial National Energy Policy Plans. That first set of TCs was subsequently used to support the analyses that were performed in 1991 by DOE for the National Energy Strategy. The TCs were updated in 1993, but until now had not been formally published and existed only in draft form.The Electric Power Research Institute (EPRI), operating on behalf of its member utilities, has conducted a program in the assessment, evaluation and advancement of renewable power technologies since the mid-1970s. In that role, EPRI has been called upon by its members, and often by the energy community in general, to provide objective information on the status and outlook for renewables in prospective electric-power applications. Toward that aim, EPRI has joined with DOE to produce this set of Renewable Energy Technology Characterizations.This joint project is one of a number of activities that DOE and EPRI are conducting under the joint DOE-EPRI Sustainable Electric Partnership entered into formally by both organizations in October 1994. It builds upon a number of activities conducted jointly by DOE and EPRI over the past two decades.1.2Objectives, Approach and ScopePurpose and Audience: In response to growing interest in renewable power technologies and the need for consistent, objective assessments of technology performance and costs, DOE and EPRI collaborated to prepare the Renewable Energy Technology Characterizations (TCs) presented in this document. Together, through this document, DOE and EPRI aim to provide for the energy community and the general public an objective picture of the status and expectations for the renewable power technologies in electric-power applications in the United States. These TCs represent a consensus between DOE and EPRI on the current status and projected development path of five renewable electricity generating technologies: biomass, geothermal, photovoltaics, solar thermal and wind. In addition, recognizing the role that storage can play in enhancing the value of some renewable power plants, a TC for storage technologies, with a strong emphasis on batteries, is included in an appendix. The TCs can serve two distinct purposes. First, they are designed to be a reference tool for energy-policy analysts and power-system planners seeking objective cost and performance data. Second, the extensive discussions of the assumptions that underlie the data provide valuable insights for R and one, employing thermal storage, is currently under test and evaluation in California. As yet, the commercial prospects for this approach cannot be accurately projected.Another approach employs parabolic dishes, either as single units or in fields, that track the sun. A receiver is placed at the focal point of the dish to collect the concentrated solar energy and heat the system’s working fluid. That fluid then drives an engine attached to the receiver. Dish systems also have potential for hybridization, although more developmental work is required to realize this potential. In contrast to the other two approaches, which are targeted at plants in the 30 MW and higher range, and which use a single turbine-generator fed by all of the solar collectors, each dish-receiver-engine unit is a self-contained electricity-generating system. Typically, these are sized at about 10 to 30 kW. Hence, a larger power plant is obtained by employing a number of these units in concert. With some interruptions due to changing market conditions, dish systems using Stirling engines have been deployed, with both public and private support, for experimental and demonstration purposes since the early 1980s. Current developmentand demonstration activities are aimed at key technical and economic issues that need to be resolved before commercial prospects can be clarified. Stirling-engine development for prospective vehicular applications is also under way. If successful, transportation sector market penetration would substantially improve the commercial outlook for solar dish- Stirling systems.The third approach employs a field of sunlight-tracking parabolic troughs that focus sunlight onto the linear axis of the trough. A glass or metal linear receiver is placed along this axis, and a working fluid is circulated through and heated in this receiver. The fluid from a field of troughs passes through a central location where thermal energy is extracted via a heat exchanger and then used to drive a conventional turbine. This configuration lends itself well to hybrid operation with fossil fuel combustion as a supplemental source of thermal energy.In the early 1980s, federal and California-state financial incentives were established to encourage the commercial deployment and use of emerging renewables. Two technologies were in a position to benefit from these incentives: solar thermal troughs and wind turbines. Trough systems were deployed on a commercial basis in the 1980s and early 1990s, and continue to operate today. In addition to the government-tax-credit incentives, these plants were partially supported by above-market energy payments that are no longer available. Hence trough systems have not been offered commercially since 1991. Should conventional energy costs rise to the above-market support levels of the late 1980s (when significant increases in oil prices were being projected), or should significant incentives for renewable energy arise in the near future, trough technology would be available to play an important role in areas with good sunlight. In addition, efforts are underway to revive this technology for use in developing countries that have urgent needs for new electric power sources, such as India and Mexico.Although the solar-thermal trough (and wind) systems fielded in the early 1980s experienced considerable technical difficulties, the overall result of the deployments of the 1980s and the associated experience and technical development was that both trough systems and wind systems (see wind discussion below) had achieved technical and commercial credibility by the early 1990s. Energy costs from these systems were approaching the competitive range for grid power. Trough-energy costs were somewhat higher than wind-energy costs; but, owing to hybridization with natural gas, the trough plants were dispatchable. Hence their energy had higher value in some instances. Wind energy, in contrast, was available only when the wind blew.Wind: As mentioned above, wind power systems progressed substantially as a result of the 1980s government incentives, with a steady trend of cost reductions throughout the 1980s. Since 1990, the cost of energy from the wind has continued to decline, due to continued deployment and to public-private development programs in the U.S. and, to an even greater extent, in Europe. Wind power is now on the verge of becoming a commercially established and competitive grid-power technology. Although expansion of the U.S. wind market has been slowed since the onset of electric-sector restructuring in 1995, the wind markets in Europe and elsewhere in the world have continued to grow, led by firms in Denmark and Germany. The growth of wind in Europe has been fueled, in part, by aggressive goals for renewable power deployment in response to strong public and political support for clean energy and growing concern over global climate change. And there are signs that the pace of wind deployment in the U.S. is again on the rise.With the exception of the Southeast, most regions of the U.S. have commercially attractive winds. In addition to wind resource quality, other issues that need to be considered, as with most commercial power plants, are transmission requirements and potential environmental impacts. Most U.S. wind facilities installed to date are wind farms with many turbines interconnected to the utility transmission grid through a dedicated substation. There is growing interest in distributed wind facilities, with a small number of turbines connected directly to the utility distribution system without a substation. Such installations account for more than half of the over 4,000 MW of wind in Europe, but the U.S. to date has little experience with this mode. Hence this document focuses on central-station wind applications.The great majority of wind power experience has been obtained with the traditional wind turbine configuration, in which the rotor revolves about a horizontal axis. In addition, several development programs of the past twenty years have focused on turbines with rotors that turn about a vertical axis (sometimes called “egg-beater” turbines). Although the case cannot be considered completely closed, the weight of experience indicates strongly that the vertical axis machines will not show a performance or commercial advantage relative to the horizontal axis machines. Hence development of the vertical axis units has all but halted, and this document focuses entirely on horizontal axis turbines.Energy Storage: Recent advances in batteries and other storage technologies have resulted in systems that can play a flexible, multi-functional role in the electricity supply network to manage power resources effectively. The current electricity market offers a number of opportunities for energy storage technologies in which storage of a few seconds to a few hours of electricity is valuable. These systems can be located near the generator, transmission line, distribution substation, or the consumer. Improved, low-maintenance, spill-proof, relatively compact lead-acid batteries are commercially available today.Energy storage systems are used beneficially today in a variety of applications. Examples include mitigation of powerquality problems and provision of back-up power for commercial/industrial customers, utility substations, and transmission-line stability. In addition, energy storage can play an important role in enabling the increased utilization of intermittent renewable energy sources such as wind and photovoltaics. In grid-connected applications, the storage system can be charged from the renewable source or from the utility grid, whichever is economically preferred.1.4Document OverviewThe five main chapters of this document correspond to five categories of renewable electricity-generating technologies -- biomass, geothermal, photovoltaics, solar thermal, and wind. Each of these five chapters has an Overview that discusses key development and deployment issues for that technology category. Each chapter has one or more Technology Characterizations (TCs); e.g., there are TCs for hydrothermal and hot dry rock systems within the geothermal technology category. Each TC was prepared in the format outlined in Figure 2. In addition, energy storage is characterized in an appendix that follows the same format. The chapter also provides estimates of levelized cost of energy using these techniques.Technology Characterization Outline 1.0 System Description: This section begins with a detailed graphic depicting key components and subsystems. A system boundary is shown, drawn around any required substation or other required grid interface equipment. The section includes a detailed discussion of the major system features, and how the system depicted in the schematic operates. 2.0 System Application, Benefits, and Impacts: This section contains a description of the applications for which the given system is designed. The motivation for developing the system is given, as is a description of the energy service provided by the system. Also delineated are the potential economic and environmental benefits and impacts. 3.0 Technology Assumptions and Issues: This section includes an explanation of current technological status and the anticipated progression of the technology through the year 2030. It also includes assumptions concerning the system being characterized, including location, commercial readiness, resource assumptions, and the energy service that the system provides. Perspectives on R&D efforts needed to ensure future progress are also presented. 4.0 Performance and Cost: This section contains the primary data table describing current (1997) and projected future (through 2030) technology cost and performance. 4.1 Evolution Overview: This subsection provides a short description of how the baseline system’s configuration, size and key components evolve over the period. 4.2 Performance and Cost Discussion: This section provides a detailed discussion to explain and justify the projections made for the technical performance and cost indicators in the table found in Section 4.0. Assumptions, methods, rationale, and references are also provided. 5.0 Land, Water, and Materials Requirements: This section contains a table and short discussion regarding the land and water requirements for the technology. It also includes a listing of any materials considered unique to the technology (e.g., cell raw materials, catalysts).6.0 References: A complete list of the literature cited is included.